Osteoporosis in children and adolescents: identifying causes and preventing future fractures
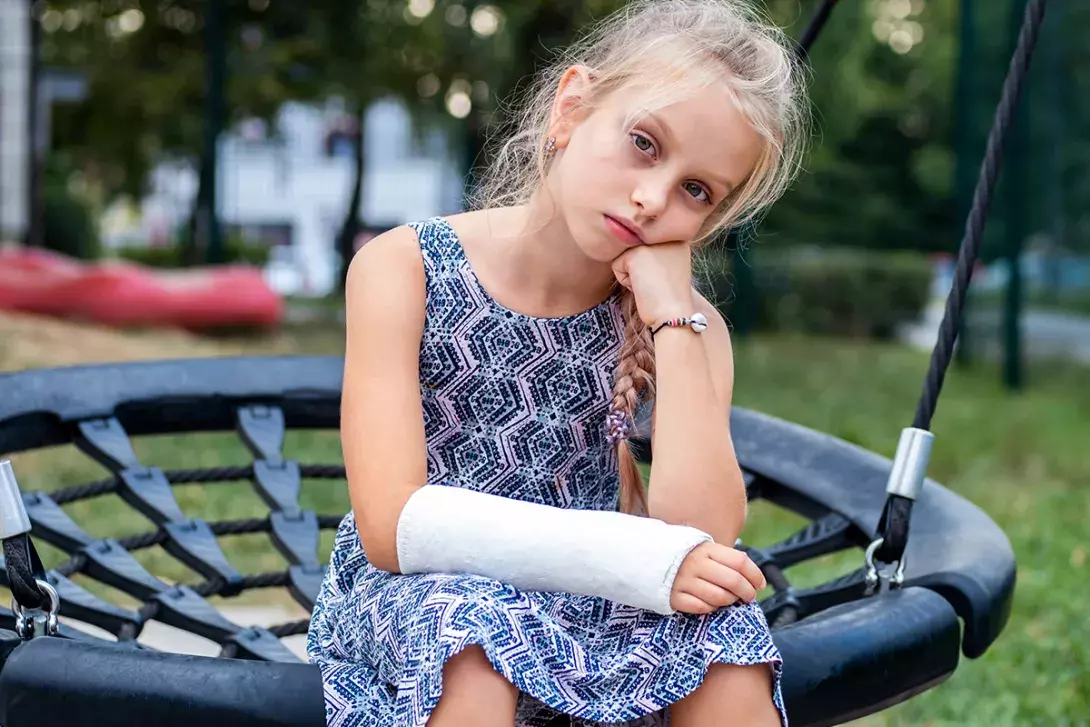
Although osteoporosis is generally considered to be associated with old age, it may present during childhood. It can result from a genetic (primary) cause or be secondary to other chronic diseases or their treatments. Fracture is the most common symptom of osteoporosis in children and adolescents, which is the same for adults; however, there are important differences and considerations when evaluating a young individual for this condition.
- Osteoporosis can present in childhood, with fractures being the most common symptom.
- The diagnosis of osteoporosis in children requires comprehensive clinical assessment and should not be made on bone densitometry alone.
- A bone health assessment should be undertaken in children who present with recurrent fractures, low trauma fractures or vertebral fractures or in those who have risk factors for developing secondary osteoporosis, such as immobility.
- First-line investigations include measurement of serum calcium, phosphate, 25-hydroxyvitamin D, parathyroid hormone and alkaline phosphatase levels, as well as imaging of the affected bone(s).
- Modifications of lifestyle factors through optimising diet and physical activity support peak bone mass accrual.
- Optimisation of peak bone mass accrual in childhood by lifestyle intervention and/or pharmacological therapy is the major preventive measure for developing osteoporosis in childhood and later in life.
- Severe paediatric osteoporosis warrants pharmacological intervention, mainly with use of bisphosphonates.
Osteoporosis, meaning porous bones, is a condition of low bone mass resulting in bone fragility. It is most often seen in post-menopausal women.1 However, osteoporosis can present in children and adolescents. Little is known in the wider medical community about osteoporosis in younger age groups. Similar to adults, osteoporosis in children and adolescents presents as fractures; however, there are important differences and considerations when evaluating a young individual for osteoporosis. This article discusses definition, presentation, assessment, causes and implications of osteoporosis in children and adolescents.
Biology of bone
To understand osteoporosis in children, it is important to understand the biology of bone in children. During growth and development, skeletal growth proceeds through the co-ordinated action of bone deposition and resorption to allow bones to expand (periosteal apposition of cortical bone) and lengthen (endochondral ossification) into their adult form. Bone mass is acquired relatively slowly during childhood. With the onset of puberty and the adolescent growth spurt in height, bone mineral accretion is rapid, reaching a peak shortly after peak height gain. This is referred to as peak bone mass, which is the amount of bone gained by the time a stable skeletal state has been attained. The higher the peak bone mass achieved, the lower the risk of osteoporosis in the future. Deficiency in bone mineral accrual during childhood can result in osteoporosis manifesting during this stage.
Definition of osteoporosis in children
The WHO first defined postmenopausal osteoporosis in 1994 as a bone mineral density (BMD) T-score of less than –2.5 at the hip, spine or distal forearm.1 Unlike in adults, diagnosis of childhood osteoporosis is not based on bone densitometry alone. In 2013 (and updated in 2019), the International Society of Clinical Densitometry defined osteoporosis in children as the presence of a clinically significant fracture history (two or more long bone fractures by 10 years of age or three or more long bone fractures by 19 years of age) and a BMD Z-score of less than –2.0.2 The exception to this definition is the presence of one or more vertebral fractures, in the absence of local disease or trauma, as a sign of osteoporosis regardless of BMD Z-scores.
Presentation
Although osteoporosis in children and adolescents may manifest as fractures, fractures are also common in this age group, especially during the adolescent growth spurt. Almost half of boys and one-third of girls will sustain one long bone fracture by the age of 18 years, with the incidence ranging from 12.0 to 36.1/1000 per year.3 The incidence of fractures peaks at 11 to 12 years of age in girls and at 13 to 14 years of age in boys, with the forearm being the most common site for fracture.4 As the bone rapidly increases in length during the growth spurt, the cortex becomes relatively thin and there is lag in mineralisation. This results in a reduced BMD, which makes the bone susceptible to fractures.5 Following an international trend, data from Western Australia show that fracture rates in youth are increasing.6
Is the fracture pathological or physiological?
If a young person presents with a fracture, it is important to take a comprehensive clinical history. Factors to evaluate are age, gender, fracture mechanism, fracture phenotype and clinical context, dietary calcium and protein intake, vitamin D status, amount of participation in weight-bearing exercise, and family history of bone fragility disorders.
The following definitions need to be considered. As per modified Landin’s criteria, which has been used in paediatric fracture studies, a low-trauma fracture is defined as a fracture resulting from a fall from standing height (less than 0.5 metres), a fall on a resilient surface (e.g. rubber or sand) from 0.5 to 3 metres, a fall from a bed or cot, playing injuries and low-impact sports injuries. A moderate trauma is considered as a fall on nonresilient surface (e.g. concrete) from 0.5 to 3 metres, fall from a bunkbed, baby being dropped to the floor by an adult, fall downstairs, fall from a bicycle and fall from swings or slides.7 Motor vehicle accidents and a fall from a height above 3 metres are considered as severe trauma. In the context of chronic illness with compromised mobility, a low trauma is defined as a fall from less than standing height at no more than walking speed.8 A vertebral fracture is defined as more than 20% loss of vertebral height using the Genant semiquantitative method, reported on spine x-ray.9
A single low-trauma long bone fracture, especially a femur fracture, could be the first manifestation of osteoporosis and should trigger further evaluation.8 A single fracture of the humerus or tibia should also be carefully evaluated for their mechanism and underlying risk factors. Forearm fractures are common in children; however, recurrent forearm fractures warrant further evaluation. Comminuted fractures, atypical fractures or displacements in low-trauma settings should be investigated. In the absence of trauma, paediatric vertebral fractures are extremely rare and are considered a sign of osteoporosis. Osteoporotic vertebral fractures may be asymptomatic.
The possibility of nonaccidental trauma should always be considered as a possible cause of fractures, especially in children under the age of 2 years. A detailed history of events leading to the fracture should be taken. If there is discrepancy in history and no plausible explanation for the fracture, this should raise the suspicion of nonaccidental trauma.10 Other clinical signs of injuries, such as bruising, marks on skin and multiple fractures that are at different stages of healing, are red flags for nonaccidental trauma.
Children with primary (genetic) bone conditions, such as osteogenesis imperfecta, may present with recurrent fractures.11 These children may have cardinal clinical signs such as blue grey sclerae, hypermobile joints, hearing impairment or dentinogenesis imperfecta. Children with a family history of recurrent fractures or premature osteoporosis could potentially have a genetic cause of osteoporosis.
Aetiology
Osteoporosis in children can be primary or secondary (Box 1 ). Osteogenesis imperfecta, a genetic condition resulting from defective collagen formation, is the prototype of primary osteoporosis. Collagen defects lead to defective bone microstructure making them brittle and prone to fractures. Recent advances in genetics have identified numerous monogenic causes of osteoporosis.12
Secondary osteoporosis can result from chronic diseases or their treatment. Following the concept of the ‘functional muscle- bone unit’ that illustrates how bone responds and adapts to muscle forces, any condition that negatively affects muscle mass or muscle forces, affects bone strength thus leading to osteopenia and ultimately osteoporosis.13,14 This is why in the context of chronic illness leading to decreased mobility, for example in people with neuromuscular disorders or in nonambulatory patients, reduced muscle mass plays a major role in the aetiology of secondary forms of osteoporosis in youth.
Glucocorticoids and some anticonvulsants or chemotherapy regimens negatively affect bone health. Treatment of chronic diseases, such as Duchenne muscular dystrophy and leukaemia, with systemic glucocorticoids has resulted in improved survival. However, this also leads to secondary osteoporosis from glucocorticoid use. Vertebral fractures can be found in one-third of children treated for leukaemia and in over 50% of boys with glucocorticoid-treated Duchenne muscular dystrophy.8 These patients require regular surveillance for bone health. Increased use of steroid-sparing agents has resulted in improved bone health, for example in patients with inflammatory bowel disease or chronic lung disease. Chronic inflammation and certain untreated conditions may negatively affect bone health (Box 1 ). Defects in mineralisation from nutritional deficiency of calcium or vitamin D cause rickets and may lead to osteoporosis due to lack in achieving peak bone mass.15 Rickets can also result from genetic causes (hypophosphataemic rickets) and disorders of vitamin D metabolism.
Assessment
A bone health assessment should be undertaken in children who present with recurrent fractures, low-trauma fractures or vertebral fractures or in those who have risk factors for developing secondary osteoporosis, such as immobility. A bone health assessment involves comprehensive clinical history-taking, including family history, personal history of fractures and related symptoms, medication use, comorbid conditions that might contribute to poor bone health and nutrition. Physical examination should cover any physical stigmata of genetic bone conditions, bony abnormalities and injury marks (Box 2 ).
First-line investigations include biochemical analysis of serum calcium, phosphate, alkaline phosphatase, parathyroid hormone and 25-hydroxyvitamin D, as well as imaging of the affected bone(s). Second-line investigations may be required depending on the results of the first-line investigations. These may include urine calcium: creatinine ratio, tubular reabsorption of phosphate and additional imaging such as skeletal survey and vertebral and bone density assessment. When to refer the child or adolescent to a paediatric bone specialist is outlined in Box 3 .
Although bone densitometry assists in clinical decision-making, it is not solely relied upon for the diagnosis of osteoporosis in children. Dual-energy x-ray absorptiometry (DXA) is the most commonly used technique for BMD assessment because of its wide availability, low radiation exposure, low cost and precision. Lumbar spine (L1 to L4) and total body less head are widely used regions for assessing BMD in children.16 Some limitations need to be considered when assessing BMD in children and adolescents. To account for growth-related changes, BMD is reported in age-adjusted Z-scores, whereas in adults it is reported as T-scores (compared with sex-adjusted peak bone mass of a young adult). DXA measures two-dimensional areal bone mineral density. Areal bone density is affected by bone size variability and therefore short children or children with pubertal delay have spuriously low bone density and tall children have spuriously high bone density on DXA compared with their age reference values. For reporting DXA Z-scores in children, height and bone age adjustments are needed.17 Before referring children or adolescents for DXA, ensure that the DXA machine has paediatric software installed and reports height-adjusted Z-scores.
Peripheral quantitative CT scan (pQCT) measures three-dimensional volumetric BMD, mainly in the forearm and lower leg. It is not affected by variability in bone size; therefore, it does not have the same limitations that apply to DXA. pQCT adds qualitative regional data on cortical and trabecular BMD and following the model of a three-point bending test, it allows the calculation of an individual’s fracture risk (stress–strain index). However, pQCT is primarily a research technique and the scanners are not widely available. It also requires appropriate reference data and expertise for undertaking and interpreting the scans.18
Treatment
The concept of peak bone mass is crucial in the assessment of paediatric bone health. Peak bone mass is the amount of bone gained by the time a stable skeletal state has been attained during young adulthood. Optimising peak bone mass during skeletal growth is paramount to preventing future fractures and osteoporosis later in life.19 Almost 20 to 40% of bone mineral accrual is determined by modifiable lifestyle factors.19 Therefore, childhood and adolescence are important periods of both vulnerability and opportunity to optimise peak bone mass. Physical activity, a healthy diet and treatment of any underlying conditions are the primary modifiable factors and the first-line measures for improving bone health and preventing osteoporosis later in life.
Exercise
Physical activity comprises any body movement produced by muscle contraction. Mechanical loading from physical activity is essential to stimulate bone modelling.20,21 Increased mechanical loads placed on bone through both impact and muscle forces, produces strain on bone, which leads to bone adaptation via osteogenic activity resulting in increased bone mass (Wolff’s law).22 Activities that subject the skeleton to large magnitude forces at rapid loading rates confer the greatest benefit for bone formation.23 Examples include activities such as jumping, hopping, skipping and those requiring multiple motor skills such as soccer, racquet sports (e.g. tennis, badminton).19 Improvements in BMD have been reported from modified/targeted exercise regimens, standing regimens, physical rehabilitation and vibration plate therapy.24
Diet
More than 99% of the body’s calcium is in the skeleton and forms the major component of bone mineral content. Calcium supplementation consistently increases gain in skeletal mass and density in children and adolescents, with vitamin D exerting its osteogenic effect via regulation of calcium levels.25,26 A positive relationship has been shown between protein intake and bone strength.27,28 Therefore, a healthy diet with adequate calories, protein and age-specific calcium and vitamin D intake is essential for optimising the health of bones.29
Management of comorbidities
Conditions that may compromise nutrition, such as malabsorption, coeliac disease and anorexia nervosa, should be addressed. Lifestyle factors such as sedentary behaviour leading to lack of exercise and an unbalanced diet are risk factors for poor bone health.
Pharmacological therapies
Severe paediatric osteoporosis warrants pharmacological intervention mainly by use of bisphosphonates. These belong to the pyrophosphate group and exert their effect by inhibiting osteoclast function, leading to reduced bone resorption with relatively increased osteoblast activity. With the ongoing growth of bones in children, this leads to an increase in bone mass and strength.30 The Bone and Mineral Working group of the Australian and New Zealand Society for Paediatric Endocrinology and Diabetes have published consensus guidelines for use of bisphosphonates in children and adolescents.31 In children, bisphosphonates are usually administered via the intravenous route, under the supervision of a specialised paediatric bone service. The duration of bisphosphonate therapy varies with the clinical condition, ongoing symptoms and change in BMD.
The use of other pharmacological agents, such as parathyroid hormone and monoclonal antibodies (e.g. denosumab), is currently limited to adults. Apart from its use in complex fractures, long-term surgical intervention (e.g. rodding) is mainly reserved for patients with severe forms of osteogenesis imperfecta.
Conclusion
Optimisation of peak bone mass accrual in childhood by lifestyle intervention and pharmacological therapy, where indicated, is the major preventive measure for developing osteoporosis later in life. Osteoporosis can present during childhood, with fractures being the most common symptom.
The diagnosis of osteoporosis in children requires a comprehensive clinical assessment and should not be made on bone densitometry alone. A bone health assessment should be undertaken in children who present with recurrent fractures, low-trauma fractures or vertebral fractures or in those who have risk factors for developing secondary osteoporosis, such as immobility. First-line investigations include measurement of serum calcium, phosphate, 25-hydroxyvitamin D, parathyroid hormone and alkaline phosphatase levels, as well as imaging of the affected bone. Lifestyle advice on healthy eating and regular physical activity for healthy bones should be part of regular consultation. ET
COMPETING INTERESTS: None.
References
1. Czerwiński E, Badurski JE, Marcinowska-Suchowierska E, Osieleniec J. Current understanding of osteoporosis according to the position of the World Health Organization (WHO) and International Osteoporosis Foundation. Ortop Traumatol Rehabil 2007; 9: 337-356.
2. Bishop N, Arundel P, Clark E, et al. Fracture prediction and the definition of osteoporosis in children and adolescents: the ISCD 2013 Pediatric Official Positions. J Clin Densitom 2014; 17: 275-280.
3. Clark EM, The epidemiology of fractures in otherwise healthy children. Curr Osteoporos Rep 2014; 12: 272-278.
4. Hedström EM, Svensson O, Bergström U, Michno P. Epidemiology of fractures in children and adolescents: Increased incidence over the past decade: a population-based study from northern Sweden. Acta Orthopaedica 2010; 81: 148-153.
5. Rauch F. The dynamics of bone structure development during pubertal growth. J Musculoskelet Neuronal Interact 2012; 12: 1-6.
6. Jenkins M, Nimphius S, Hart NH, et al. Appendicular fracture epidemiology of children and adolescents: a 10-year case review in Western Australia (2005 to 2015). Arch Osteoporos 2018; 13: 63.
7. Clark EM, Ness AR, Tobias JH. Bone fragility contributes to the risk of fracture in children, even after moderate and severe trauma. J Bone Mineral Res 2008; 23: 173-179.
8. Ward LM, Weber DR, Munns CF, Hogler W, Zemel BS. A contemporary view of the definition and diagnosis of osteoporosis in children and adolescents. J Clin Endocrinol Metab 2020; 105: e2088-e2097.
9. Genant HK, Wu CY, Kuijk CV, Nevitt MC. Vertebral fracture assessment using a semiquantitative technique. J Bone Miner Res 1993; 8: 1137-1148.
10. Pereira EM. Clinical perspectives on osteogenesis imperfecta versus non-accidental injury. Am J Med Genet C Semin Med Genet 2015; 169: 302-306.
11. Joshi KK, Siafarikas A, Prince R. The natural history of symptomatic fractures in children and adolescents with osteogenesis imperfecta type 1: a cohort study from Western Australia. JBMR Plus 2023; e10782.
12. Mäkitie RE, Costantini A, Kampe A, Alm JJ, Makitie O. New insights into monogenic causes of osteoporosis. Front Endocrinol 2019; 10: 70.
13. Schoenau E, Frost H. The "muscle-bone unit" in children and adolescents. Calcif Tissue Int 2002; 70: 405-407.
14. Frost HM. Bone "mass" and the "mechanostat": a proposal. Anat Rec 1987; 219: 1-9.
15. Diaz-Thomas A, Iyer P. Global health disparities in childhood rickets. Endocrinol Metab Clin North Am 2023; 52: 643-657.
16. Weber DR, Boyce A, Gordon C, et al. The utility of DXA assessment at the forearm, proximal femur, and lateral distal femur, and vertebral fracture assessment in the pediatric population: 2019 ISCD official position. J Clin Densitom 2019; 22: 567-589.
17. Zemel BS, Leonard MB, Kelly A, et al. Height adjustment in assessing dual energy x-ray absorptiometry measurements of bone mass and density in children. J Clin Endocrinol Metab 2010; 95: 1265-1273.
18. Adams, JE, Engelke K, Zemel BS, Ward KA, International Society of Clinical Densitomtry. Quantitative computer tomography in children and adolescents: the 2013 ISCD Pediatric Official Positions. J Clin Densitom 2014; 17: 258-274.
19. Weaver CM, Gordon CM, Janz KF, et al. The National Osteoporosis Foundation’s position statement on peak bone mass development and lifestyle factors: a systematic review and implementation recommendations. Osteopor Int 2016; 27: 1281-1386.
20. Specker B, Binkley T. Randomized trial of physical activity and calcium supplementation on bone mineral content in 3- to 5-year-old children. J Bone Miner Res 2003; 18: 885-892.
21. Duckham, RL, Baxter-Jones ADG, Johnston JD, Vatanparast, H, Cooper D, Kontulainen S. Does physical activity in adolescence have site‐specific and sex‐specific benefits on young adult bone size, content, and estimated strength? J Bone Miner Res 2014; 29: 479-486.
22. Forwood M, Turner CJB. Skeletal adaptations to mechanical usage: results from tibial loading studies in rats. Bone 1995; 17: S197-S205.
23. Weeks BK, Beck BR. The BPAQ: a bone-specific physical activity assessment instrument. Osteopor Int 2008; 19: 1567-1577.
24. Ward K, Alsop C, Caulton J, Rubin C, Adams J, Mughal Z. Low magnitude mechanical loading is osteogenic in children with disabling conditions. J Bone Miner Res 2004; 19: 360-369.
25. DeLuca HF, Sicinski RR, Tanaka Y, Stern PH, Smith CM. Biological activity of 1, 25-dihydroxyvitamin D2 and 24-epi-1, 25-dihydroxyvitamin D2. AM J Physiol 1988; 254: E402-E406.
26. El-Hajj Fuleihan G, Nabulsi M, Tamim H, et al. Effect of vitamin D replacement on musculoskeletal parameters in school children: a randomized controlled trial. J Clin Endocrinol Metab 2006; 91: 405-412.
27. Bounds W, Skinner J, Carruth BR, Ziegler P. The relationship of dietary and lifestyle factors to bone mineral indexes in children. J Am Diet Assoc 2005; 105: 735-741.
28. Alexy U, Remer T, Manz F, Neu CM, Schoenau E. Long-term protein intake and dietary potential renal acid load are associated with bone modeling and remodeling at the proximal radius in healthy children. Am J Clin Nutr 2005; 82: 1107-114.
29. Siafarikas A, Simm P, Zacharin M, et al, Global consensus on nutritional rickets: Implications for Australia. J Paediatr Child Health 2020; 56: 841-846.
30. Rauch F, Plotkin H, Zeitlin L, Glorieux FH. Bone mass, size, and density in children and adolescents with osteogenesis imperfecta: effect of intravenous pamidronate therapy. J Bone Miner Res 2003; 18: 610-614.
31. Simm PJ, Biggin A, Zacharin MR, et al. Consensus guidelines on the use of bisphosphonate therapy in children and adolescents. J Paediatr Child Health 2018; 54: 223-233.